Hydrogen in the Energy Transition
In this cover the basics article, we answer some frequently asked questions about hydrogen and we explore how it contributes to the energy transition.
Achieving the ambitions of the EU Green Deal will require innovation across virtually all areas of the economy. One tool which is believed by many to be useful in addressing tricky decarbonisation issues in multiple sectors is clean hydrogen.
In this ‘Cover the basics’ article, we aim to map the contemporary hydrogen landscape by responding to six common questions. What is hydrogen? What are the current and potential uses of hydrogen? How is it produced? What do hydrogen supply chains look like? Which strategies and laws are aimed at supporting a clean hydrogen economy in the EU? How is the uptake of hydrogen being financed?
What is hydrogen?
Confusingly, we can talk about hydrogen in both its atomic and molecular forms. The hydrogen atom is by a huge margin the most common atom in the known universe, making up roughly three-quarters of its mass (see Figure 1). However, hydrogen in molecular form, ‘H2,’ i.e. two hydrogen atoms bonded together, is scarce and is typically found in combination with other atoms, like oxygen in the case of water (H20) or carbon in the case of methane (CH4).
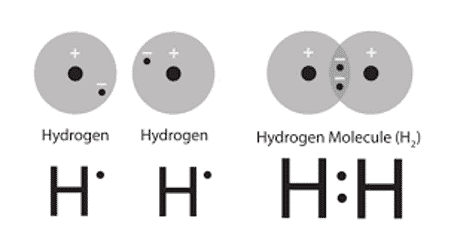
Hydrogen molecules are the focus of this article. At room temperature, hydrogen molecules are an odourless colourless gas with the lowest density of any gas. As we typically do not extract gaseous hydrogen as we can with some other energy vectors, we must liberate it from other products such as methane, water, coal and biomass. This can be done through a variety of processes, which we will explore later.
What current and potential future uses are there for hydrogen?
We focus on two broad applications of hydrogen (i) as an industrial feedstock and reductant, and as an (ii) energy vector in the power system. The EU currently consumes roughly 8 million tonnes (MT) of hydrogen per year, equivalent to around 2% of energy demand.
More than 90% of the existing demand for hydrogen in the EU is related to industrial processes, in which hydrogen is a feedstock and a reductant.
- Removing impurities during the crude oil refining process (~50% of total).
- Chemical plants use hydrogen as a feedstock (i.e. raw material) to produce a wide variety of products including ammonia (NH3), methanol (CH3OH), fertilisers, household products and industrial solvents (~40% of total).
- Steel production from direct reduced iron (DRI) and other industrial uses, e.g. as a blanketing gas and a coolant (~5%).
- Other (~5%)
In a decarbonised future we can expect that demand for crude oil will drop considerably due to electrification, whilst the EU is also hoping to reduce fertiliser use by 20% by 2030, cutting another substantial chunk of hydrogen demand. What remains of current fossil hydrogen demand will need to be replaced with more sustainably produced hydrogen.
In recent years hydrogen has also caught the attention of other new sectors, as a means of ‘in-direct electrification’, i.e. transforming clean electrons into molecules, giving it different properties that make it useful in a wider range of applications.
Four new categories of energy sector applications for hydrogen moving forward:
- Buildings (e.g. space heating, water heating, cooking);
- Industry (e.g. high-temperature steam in the glass and cement industries);
- Mobility (e.g. for heavy-duty vehicles, derivatives for aviation);
- Electricity generation and grid balancing (e.g. seasonal storage of electricity – stored as hydrogen – and electricity generation during peak loads from hydrogen-based gas turbines or fuel cells).
It is worth noting that virtually all these new uses are still subject to a technology race against other decarbonisation solutions. Clean hydrogen can be useful in a huge range of applications, but it is rarely the optimal tool for the job. We can say with relative certainty that hydrogen will continue to be needed in the chemicals sector, for DRI, and to produce a minimum of synthetic fuels for heavy transport. In the EU this could be less than half of the current 8MT of consumption, any additional demand uses are subject to a technology race, or strategic technology picking on the part of governments, policymakers, and industry leaders.
How is hydrogen produced?
The key reason why clean hydrogen is rarely the optimal tool for decarbonisation is that it is quite inefficient to produce. As discussed earlier, we typically liberate hydrogen for other products (H20, CH4, etc), and breaking those chemical bonds incurs significant energy losses versus using the energy source directly (methane, coal, electricity, biomass). Currently, hydrogen is produced either at dedicated facilities (~60%) or as a by-product of other energy-intensive chemical production processes, e.g. chlorine.
Below we explore the main ways that hydrogen is produced at dedicated facilities and how clean they are (Figure 2).
- Black – produced via gasification of ‘black’ coal.
- Brown – produced via gasification of ‘brown’ coal.
- Grey – produced via thermochemical conversion of fossil gas, either Auto-thermal Reforming (ATR) or Steam Methane Reforming (SMR).
- Blue – produced via ATR or SMR of fossil gas, with the addition of carbon capture (use) and storage (CCUS).
- Turquoise – produced via pyrolysis of methane gas (fossil or bio) driven by electricity (non-renewable or renewable). More info here.
- Pink – produced via electrolysis of water, utilising electricity of nuclear origin.
- Green -produced via electrolysis of water, driven by renewable electricity.
- Yellow – produced via electrolysis of water, utilising grid electricity.
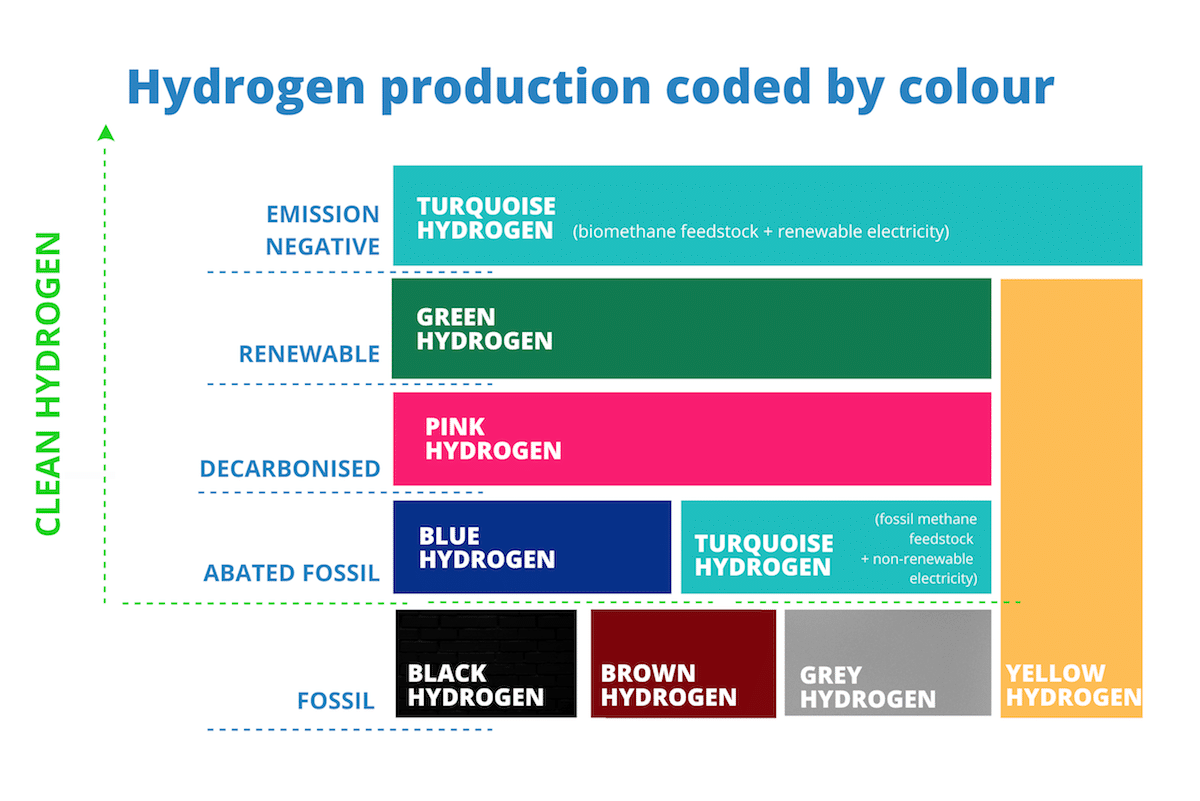
Currently, only 0.3% of the hydrogen produced in the EU is of renewable origin, almost all production is ‘grey’, contributing roughly 2% of our total emissions. The story globally is similar, albeit with a higher utilisation of coal based (black or brown) hydrogen in East Asia. It is important to keep in mind that lifecycle greenhouse gas (GHG) emissions also depend on emissions associated with supply chains. For example, the GHG emissions of the natural gas supply chain can be significant and highly variable due to methane leaks. Although emissions from electricity supply chains can be negligible if the electricity is from renewable sources, fossil origin electricity (i.e. from coal or gas power plants) can produce electrolytic (‘yellow’) hydrogen with higher emissions than grey hydrogen. This is one of the challenges in the certification of hydrogen.
What do hydrogen supply chains look like?
The vast majority of hydrogen currently consumed is produced at the point of consumption or nearby, typically connected via a short private network. However, different forms of clean hydrogen require various conditions, such as abundant renewable electricity or suitable geological conditions for the storage of CO2. In these new value chains, there is a requirement for storage and transport infrastructure to bring the hydrogen to demand centres, or for demand centres to relocate closer to the supply. In this article, we focus on the former.
Hydrogen can be stored in different ways depending on its state (gaseous or liquid). For example, gaseous hydrogen can be stored in salt caverns or pressurised tanks. Geological storage is far cheaper than above-ground storage in manmade infrastructure.
There are two transportation options for hydrogen, with very different infrastructural implications:
- Pipelines (gaseous): transporting either pure hydrogen or hydrogen blended into the existing methane network;
- Shipping (liquid): either as hydrogen (once cooled to -252°C), in the form of ammonia or methanol, or embedded in a liquid organic hydrogen carrier (LOHC).
Transport costs can vary greatly according to the volume of hydrogen demand and the distance required for it to be transported. Repurposed gas pipelines are widely believed to be the cheapest method of transporting hydrogen under most circumstances, where available. Even where new pipelines would be required, pipeline technology remains typically the more cost-effective option, provided a minimum volume can be reached. This is largely due to the equipment, processing, and energy required to convert gaseous hydrogen either into liquid form, embed in a LOHC, or transform into a carrier, and then reverse these processes upon delivery. These complexities are compounded by equipment costs and a shortage of skills for handling such a cold and volatile product at scale. Nevertheless, for small quantities, and particularly where there is alignment between the shipping medium and the final demand (e.g. ammonia kept as ammonia), it can be a cost-effective and practical solution, particularly whilst pipeline infrastructure is still being built. See Figure 3 below for a visual comparison of technology options and costs, also relative to a high-voltage direct current (HVDC) electricity cable.
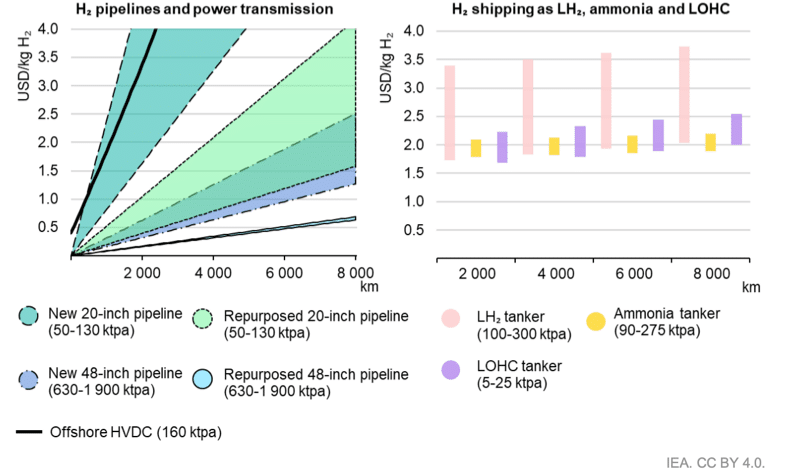
Blending relatively low volumes of hydrogen into the natural gas grid (<5%~) is an option that is already available with current infrastructure, and it has been facilitated in the ‘Hydrogen and Decarbonised Gas Market Package’ (HDGMP). This approach has the advantage of providing immediate offtake options for producers, and potentially very marginally reducing the emission intensity of gas in the network. Nevertheless, the resulting gas mix will be of lower energy density than pure methane gas, create equipment interoperability issues beyond very low hydrogen volumes, be hugely energy inefficient when accounting for the energy input for hydrogen production, and will create challenges at end use, such as ‘de-blending’. This market evolution will mark a clear departure from the current configuration of gas supply chains, which almost exclusively transport pure methane or pure hydrogen.
What strategies and laws are most relevant to clean hydrogen in the EU?
As part of the European Green Deal, the European Commission’s complementary strategies ‘A hydrogen strategy for a climate-neutral Europe’ (Hydrogen Strategy) and ‘An EU Strategy for Energy System Integration’ (ESI Strategy) both identify ‘clean’ hydrogen and other synthetic fuels as necessary to reach full decarbonisation.
The ESI Strategy describes the “use of renewable and low-carbon fuels, including hydrogen, for end-use applications where direct heating or electrification are not feasible, nor efficient or have higher costs” as some of the important uses of hydrogen in the context of energy system integration. Moreover, electrolysers are one of the key tools for sector coupling between renewable electricity and renewable gas networks. This approach is consistent with the logic of using primarily electrolytic renewable hydrogen as ‘in-direct electrification’ where electrons are not well suited.
The Hydrogen Strategy identifies cumulative investment needs and policies to promote the development of value chains for low-carbon and renewable hydrogen. These aims are broken down into the following three phases:
- Phase 1 (from 2020 to 2024): installation of at least 6 gigawatts (GW) of electrolyser capacity in the EU by 2024, corresponding to production of up to 1 million tonnes of renewable hydrogen. This aim was not realised.
- Phase 2 (from 2025 to 2030): installation of at least 40 GW of electrolyser capacity in the EU by 2030, with a further 40GW installed in the neighbourhood region (Ukraine and North Africa) – corresponding to ~6MT of renewable hydrogen in the EU.
- Phase 3 (from 2030 onwards and towards 2050): renewable and low-carbon hydrogen technologies should reach maturity and be deployed on a large scale.
The ‘REPowerEU’ documents released in March and May 2022 in response to the Russian invasion of Ukraine increased these targets considerably, to leverage hydrogen and other clean molecules to enhance energy security. The total production and import target for renewable and low-carbon hydrogen rose to 20 million tonnes by 2030, in the hope that this could replace 27 billion cubic metres (BCM) of Russian gas. The Communications also emphasise the need to ramp up the development of corresponding infrastructure, including storage, and promised expedited assessment and processing of hydrogen projects under the ‘Important Projects of Common European Interest’ (IPCEI) and state aid procedures.
The ‘Hydrogen and Decarbonised Gas Market Package’ (HDGMP) is perhaps the most important development since the ESI and Hydrogen Strategies of 2020, particularly in terms of setting the incentives and market conditions for the uptake of hydrogen and other clean molecules. The HDGMP is the fourth iteration of comprehensive legislation in the sector, following the ‘Third Energy Package’ of 2009 and it formally integrates hydrogen into the normal functioning of the natural gas market. The Package proposes to recast the Regulation on conditions for access to natural gas transmission networks (715/2009) (‘Gas Regulation’) and the Directive on common rules for the internal market for natural gas (2009/73) (‘Gas Directive’).
The core aims of the updates are to (I) establish the conditions for facilitating rapid and sustained uptake of renewable and low-carbon gases, (II) improve market conditions and increase engagement of gas consumers, (III) better account for contemporary security of supply concerns, (IV) address price and supply concerns at the Union level and (V) recalibrate the structure and composition of regulatory bodies.
For hydrogen specifically, the package attempts to (I) define more clearly the different forms of clean hydrogen, (II) provide incentives for the uptake of clean hydrogen, and (III) propose a specific framework for the management and planning of a clean hydrogen sector.
- I. Regarding definitions, Articles 2(10 & 12) and 8 of the Gas Directive offer definitions of ‘low-carbon hydrogen’ and ‘low-carbon gases’ more widely, indicating a “greenhouse gas emission reduction threshold of 70%.” compared to the fossil fuel comparator EF(t) set out in Annex V of Directive (EU) 2018/2001. The Directive also refers to Article 2 of REDII for classifications of ‘renewable gas,’ ‘low-carbon gas,’ ‘low-carbon fuel’ and ‘renewable fuels of non-biological origin’ (RFNBOs).
A specific methodology for calculating and defining the thresholds and conditions for RFNBOs were defined in subsequent Delegated Acts, with a commitment to do the same for ‘low-carbon’ gases within 12 months of the Directive’s adoption, as per Article 83.
- II. Regarding incentives, renewable gas will receive a 100% discount and low-carbon gas will receive a 75% discount from various entry and exit tariffs as per Article 16 of the Gas Regulation. Moreover, for the first year after entry into force of the Regulation, tariffs will not be chargeable for transmission of renewable gases across interconnection points between Member States, with a 75% discount for low-carbon gas.
- III. Regarding management and planning of the network, first, an entity for European Distribution System Operators (DSOs) will be set up. Full details of the scope and role of the entity can be found in Articles 36 and 37 of the Gas Regulation.
Second, a network association will be established for hydrogen network operators, ‘The European Network for Network Operators of Hydrogen’ (ENNOH) (Articles 21-24, 26-29, 40-47). ENNOH’s tasks will include contributing to relevant network codes and Union-wide non-binding ten-year network development plans (TYNDPs) for the hydrogen sector.
Third, a 2% blend of hydrogen into the natural gas network must be accepted and facilitated at cross-border interconnection points (Article 19, Regulation). However, it is specified that blending hydrogen into the natural gas grid should be considered a ‘last-resort’ use case, given the inefficiency of this application (43).
How is the uptake of hydrogen being financed?
The funding landscape for clean hydrogen in Europe is very complex, as the value chains can touch everything from technology, research and innovation to major infrastructure, energy credits, and much more besides. The diagram below illustrates where hydrogen is included in the scope of available European funds, only in the ‘hydrogen specific funds’ is all the money earmarked for hydrogen. There are also several national-level schemes and private-public blended finance schemes.
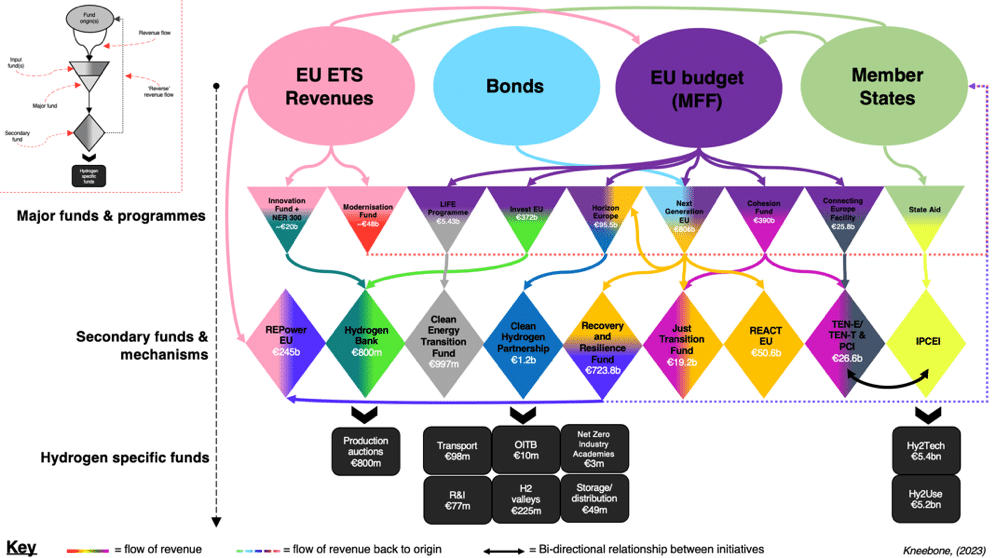
Since the mid-2010’s financial support mechanisms have moved away from research and innovation, support for pilot projects, and towards production credits. This can be seen in the mandate of the Fuel Cells and Hydrogen 2 Joint Undertaking (FCH2JU) from 2014 – 2020 which was focused on research, technological development, and demonstration, relative to the Clean Hydrogen Joint Undertaking (CHJU) that succeeded it. Although the CHJU is still focused on innovation, the projects are typically more mature, focused on ‘market entry’ and ‘deployment’, rather than proof of concept.
Moreover, in early 2024 the EU conducted its first auction for renewable hydrogen production credits, under the Innovation Fund backed ‘EU Hydrogen Bank’. The first round of auctions had a budget of €800m and attracted 132 bids. Given that the Bank has various pre-qualification criteria and an obligation that projects enter operation within 5 years of signing the support grant, the high level of interest suggests that there might be a critical mass of production coming online before 2030. Nevertheless, given a support ceiling of €4.5/kg, the €800m could return as little as 177,000t of hydrogen, in the most pessimistic case. However, the competitive nature of the auction means that the real return will likely be much higher than that, and a second round of auctions could have a budget of up to €2.2bn, which would ensure much larger volumes.
Another key support mechanism in the EU are IPCEIs which are specific Member State funded projects with a wider EU value. To date, there have been three rounds of IPCEI projects for hydrogen, totalling more than €15bn in support. To receive an exemption to state aid rules, projects need to demonstrate their ‘common value’ for the EU, or at least to multiple Member States, nevertheless, projects remain heavily concentrated within a handful of Member States who have the fiscal capacity to invest. The most recent round ‘Hy2Infra’ included electrolyser projects, transmission and distribution pipelines, import terminals, and storage facilities, which is emblematic of the EU’s attempts to support all segments of the value chain in parallel. For a more detailed breakdown, see here.
References
If you still have questions or doubts about the topic, do not hesitate to contact one of our academic experts:
James Kneebone, Ronnie Belmans
Relevant links
Opinion | A first look at the EU Hydrogen and Decarbonised Gas Markets Package by James Kneebone
Opinion | A first look at REPowerEU: The European Commission’s plan for energy independence from Russia by James Kneebone
Publication | Florence school of regulation : cost-effective decarbonisation study 2022 – Technical Report AUTHORS: Christopher Jones, James Kneebone, Andris Piebalgs
Publication | Molecules : indispensable in the decarbonized energy chain AUTHORS: BELMANS Ronnie, VINGERHOETS Pieter
Highlights from the FSR online debate| Between Green and Blue: a debate on Turquoise Hydrogen
Highlights from the FSR online debate | What needs to be done to deliver on enhanced hydrogen ambition in the EU? A timely debate between key stakeholders
Publication | Hydrogen technology summary AUTHORS: Ivana Čeković, Ilaria Conti, Christopher Jones, Andris Piebalgs
Publication | Green hydrogen : bridging the energy transition in Africa and Europe AUTHORS: Swetha RaviKumar Bhagwat, Maria Olczak